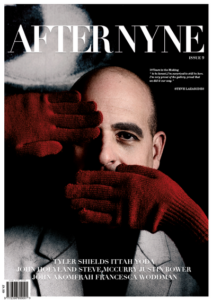
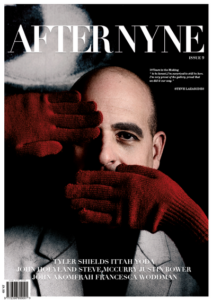
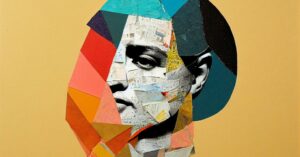
The subjective experience of thinking
October 16, 2023
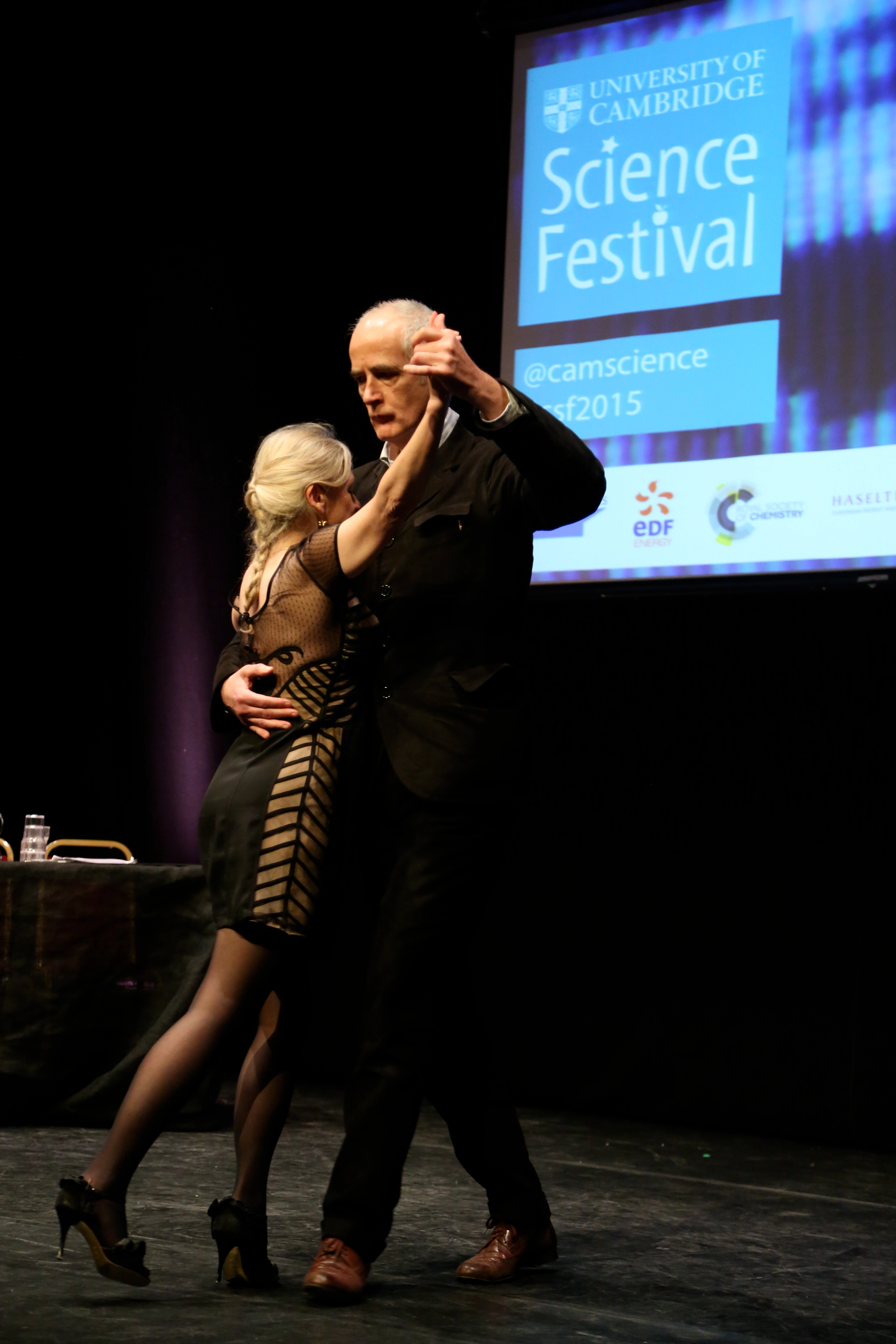
What The Captured Thought got up to at the Famelab
May 30, 2023
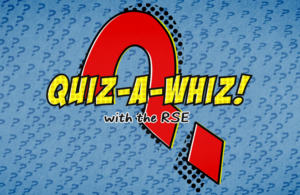
Quiz-A-Whiz!~ from The Royal Society of Edinburgh.
May 30, 2023
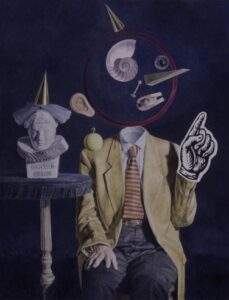
The Captured Thought~ Seven myths of memory
May 30, 2023
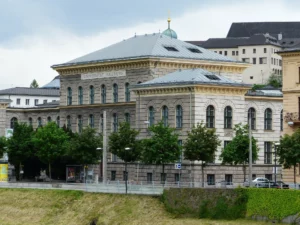